Development and calibration of a snow-hydro-karst-gravimetric model for high-alpine catchments
Supervisor: Karsten SCHULZ
Project assigned to: Roberta FACCHINETTI
Within high-alpine regions, snowpack and snow water equivalent (SWE) represent a major seasonal storage and flux component (Barnett et al., 2005). In alpine environments, where snow accumulation and melt control the timing and magnitude of runoff, accurate spatiotemporal monitoring and modeling of SWE is crucial for regional water resource management, and for anticipating the effects of climate change on hydrological systems (Beniston et al., 2018; Immerzeel et al., 2020; Viviroli et al., 2007). Karstified alpine catchments, such as those in the Zugspitze Massif, pose particular challenges due to their complex subsurface structures and high heterogeneity.
The study of my thesis will integrate snow-karst-hydrological modelling with terrestrial gravimetry to improve water balance estimation in such environments. Superconducting gravimeters (SGs), offer continuous, high-frequency measurements that, to the order of nm/s², are indicative of changes in total water storage, resulting from mass redistribution associated with hydrological processes (Güntner et al., 2017). Recent studies in alpine (Voigt et al., 2021; Koch et al., 2024) and karst (Mouyen et al., 2019; Watlet et al., 2020) environments have demonstrated their capability to capture snowpack accumulation, recharge events, and even evapotranspiration signals.
The high-alpine Zugspitze region, located at the German-Austrian border, offers a unique setting for advancing snow-hydro-karst-gravimetric research. At the top of the mountain, the Zugspitze Geodynamic Observatory Germany (ZUGOG) is located, where an SG has been operating since autumn 2018 by GFZ Potsdam. According to Voigt et al. (2021), gravity residuals already from the first years of observations show a high sensitivity to Snow Water Equivalent (SWE), exhibiting similar seasonal patterns. The study area is well-instrumented, with long-term hydrometeorological data (Weber et al., 2021; DWD, 2024) and three monitored catchments: Partnach Spring, Bockhütte, and Hammersbach.
The whole Zugspitze area is heavily karstified, and particular geological conditions allow the Partnach Spring catchment to be a closed hydrological karst system acting as a natural lysimeter, making it ideal for water balance investigations (Wetzel, 2004).
The overarching goal is to advance the understanding of water storage and flux partitioning in high-alpine karst environments by integrating snow-karst-hydrological modelling with terrestrial gravimetry, and is structured in two successive phases:
Phase I: Coupled snow and karst hydrological modelling
The first objective is to develop a coupled modelling framework that combines a physically-based snow model with a conceptual karst-hydrological model. Conceptual models are often favored over physically based models in karst regions, particularly in areas with limited subsurface information. The complex and heterogeneous nature of karst systems, characterized by features such as underground channels, fissures, and sinkholes, would arise too many uncertainties for physically based modeling reducing their reliability and predictive value (Hartmann et al., 2014). On the other hand, snow is not only a dominant seasonal water storage component in alpine systems but also plays a key role in controlling the timing and magnitude of recharge to karst aquifers (Beniston et al., 2018; McGlynn et al., 2004). Therefore, accurate representation of snow dynamics, particularly snow water equivalent (SWE), and its spatiotemporal distribution, is therefore essential for modelling internal catchment processes (Dozier et al., 2016; Dong, 2018).
Key research questions (Phase I):
- What methods enable a good representation of runoff in simple hydrological models within karstified high-alpine catchments?
- How can hydro-karst modeling be refined? Does incrementally increasing the snow complexity lead to an improvement in accuracy and capturing the seasonality well for high-alpine catchment simulations?
- How can detailed, physically-based snowpack models such as SNOWPACK/Alpine3D be effectively integrated with conceptual karst-hydrological models to simulate the spatiotemporal variability of recharge in high-alpine karst environments?
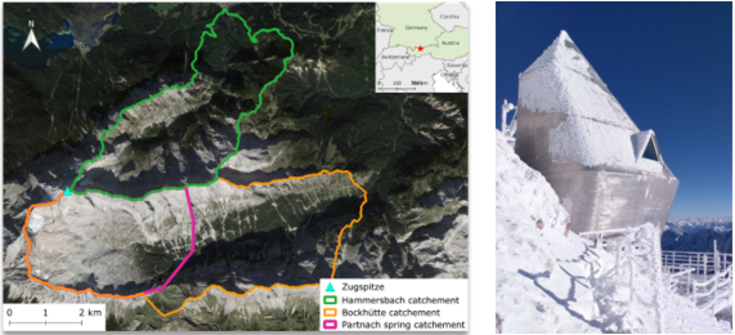
Figure 1: The Zugspitze study area with the three gauged catchments (source: Google Satellite)
Figure 2: The Zugspitze Geodynamic Observatory Germany (ZUGOG) operated by GFZ Potsdam (Photo: Christian Voigt, GFZ)
Phase II: Forward modelling of gravity signals
The second objective is to develop a forward operator that translates simulated water mass changes into gravity signals. This operator will simulate gravity changes attributable to mass fluctuations in various hydrological components, such as SWE, groundwater storage, evapotranspiration, and long-term changes in the cryosphere (e.g., glaciers, dead ice, and permafrost), enabling a direct comparison between hydrological model outputs and terrestrial gravity observations. This method is based on Newton’s law of universal gravitation, which states that differences in gravitational acceleration can be detected over time as a result of changes in hydrological mass storage (Van Camp et al., 2017; Crossley et al., 2013).
The forward operator will calculate the gravitational effect of such storage variations over time, considering their spatial distribution and proximity to the gravimeter.
Key research questions (Phase II):
What is the influence of different hydrological fluxes and storages (such as precipitation, snow accumulation, rain events, and glacier changes) on the gravimeter signal? Can we extract the relative contributions of each of these?
How can modelled SWE and groundwater storage changes be translated into high-resolution synthetic gravity signals?
How well does the forward model reproduce the observed signal characteristics recorded at Zugspitze?
How can gravimetric data enhance model calibration and reduce uncertainties?
Can terrestrial gravimetry serve as a reliable constraint to reduce uncertainty in coupled snow-karst hydrological model predictions?
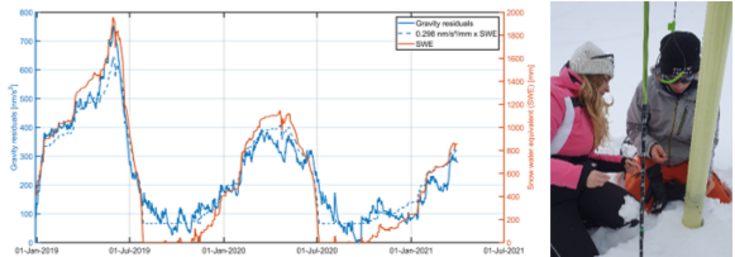
Figure 3: Gravity residuals (solid blue), snow water equivalent (solid orange) from LWD station at Zugspitzplatt and SWE multiplied with the estimated regression factor of 0.298 nm s−2 mm−1 between gravity residuals and snow water equivalent (dashed blue line) (Voigt et al., 2021).
Figure 4: Taking density sample with a snow density sampler (i.e. Dolphi device) during the field measurement campaign at the Zugspitzplatt in winter 2024.
References
Barnett, T., Adam, J. & Lettenmaier, D. Potential impacts of a warming climate on water availability in snow-dominated regions. Nature 438, 303–309 (2005).
Beniston, M., Farinotti, D., Stoffel, M., Andreassen, L.M., etc. & Vincent, C. (2018) The European mountain cryosphere: a review of its current state, trends, and future challenges. Cryosphere 12, 759-794.
Crossley, D. J., et al. (2013). A review of the global superconducting gravimeter network (GGP). Surveys in Geophysics.
Dong, C.Y. (2018) Remote sensing, hydrological modeling and in situ observations in snow cover research: A review. J. Hydrol. 561, 573-583.
Dozier, J., Bair, E.H. & Davis, R.E. (2016) Estimating the spatial distribution of snow water equivalent in the 22 world's mountains. Wires Water 3, 461-474.
Güntner, A., Reich, M., Mikolaj, M., Creutzfeldt, B., Schroeder, S. and Wziontek, H. (2017) Landscape-scale water balance monitoring with an iGrav superconducting gravimeter in a field enclosure. HESS 21, 3167-3182.
Hartmann, A., Goldscheider, N., Wagener, T., Lange, J. & Weiler, M. (2014) Karst water resources in a changing world: Review of hydrological modeling approaches. Reviews of Geophysics, 52(3), pp.218-242.
Immerzeel, W.W., Lutz, A.F., Andrade, M., Bahl, A., Biemans, H., Bolch, T., Hyde, S., etc. & Baillie, J.E.M. (2020) Importance and vulnerability of the world's water towers. Nature 577, 364-+.
Koch, F., Gascoin, S., Achmüller, K., Schattan, P., Wetzel, K.F., Deschamps‐Berger, C., Lehning, M., Rehm, T., Schulz, K. and Voigt, C., 2024. Superconducting gravimeter observations show that a satellite‐derived snow depth image improves the simulation of the snow water equivalent evolution in a high alpine site. Geophysical Research Letters, 51(24), p.e2024GL112483.
McGlynn, B.L., McDonnell, J.J., Seibert, J. & Kendall, C. (2004) Scale effects on headwater catchment runoff timing, flow sources, and groundwater-streamflow relations. Water Resour. Res. 40.
Mouyen, M., Longuevergne, L., Chalikakis, K.,Mazzilli, N., Ollivier, C., Rosat, S., Hinderer, J. & Champollion, C. (2019) Monitoring of groundwater redistribution in a karst aquifer using a superconducting gravimeter. E3S Web of Conferences 88, 03001.
Van Camp, M., de Viron, O., Pajot‐Métivier, G., Casenave, F., Watlet, A., Dassargues, A., & Vanclooster, M. (2016). Direct measurement of evapotranspiration from a forest using a superconducting gravimeter. Geophysical Research Letters, 43(19), 10-225.
Viviroli, D., Durr, H.H., Messerli, B., Meybeck, M. & Weingartner, R. (2007) Mountains of the world, water towers for humanity: Typology, mapping, and global significance. Water Resour. Res. 43.
Voigt, C., Schulz, K., Koch, F., Wetzel, K.F., Timmen, L., Rehm, T., Pflug, H., Stolarczuk, N., Förste, C. & Flechtner, F. (2021) Technical note: Introduction of a superconducting gravimeter as novel hydrological sensor for the Alpine research catchment Zugspitze. Hydrol. Earth Syst. Sci. 25, 9, 5047-5064.
Watlet, A., Van Camp, M., Francis, O., Poulain, A., Rochez, G., Hallet, V., Quinif, Y. & Kaufmann, O. (2020) Gravity Monitoring of Underground Flash Flood Events to Study Their Impact on Groundwater Recharge and the Distribution of Karst Voids. Water Resour. Res. 56.
Weber, M., Koch, F., Bernhardt, M. & Schulz, K. (2021) The evaluation of the potential of global data products for snow hydrological modelling in ungauged high-alpine catchments. HESS, 25, 2869-2894.
Wetzel, K.-F. (2004) On the hydrology of the Partnach area in the Wetterstein mountains (Bavarian Alps). Erdkunde 58, 172-186.