Overview project results
The advanced countryside species-area relationship model
We developed a novel approach to link socio-economic metabolism, specifically the consumption of biomass products, to land use (LU) and the resulting impact on biodiversity, suitable to calculate the biodiversity footprint of urban systems in high product resolution. Our approach is based on the countryside species-area relationship (cSAR; Pereira et al. (2014)). We applied the model to terrestrial vertebrate data on a fine spatial resolution of 5 x 5 arc minutes, based on LU-data featuring 45 LU types and developed a novel approach to include spatially explicit, continuous LU-intensity descriptors at the same resolution in order to separate biodiversity effects of land conversion (i.e. the replacement of pristine ecosystems by various LU types) from those of LU-intensity (i.e. the effects of management without changing the LU type) in both converted (such as cropland) and unconverted ecosystems (such as natural grazing land and forests subject to LU). For this we linked model coefficients from Newbold et al. (2015), who analyze local assemblage data from the PREDICTS database (Hudson et al. 2014) with spatially explicit LU-intensity indicators around the base year 2010. In order to take the multidimensional nature of land-use intensity, as well as the large data uncertainties related to land-use intensity information into account, we developed two intensity indicator sets. One set is based on the human appropriation of net primary production (HANPP), a comprehensive, systemic metric of LU-intensity which is based on measuring the effect of LU on the availability of annual biomass flows (trophic energy) in the ecosystem and is calculated for all LU-types (Haberl et al. 2014). The alternative set of intensity indicators combines input and output metrics of agricultural LU systems, such as fertilizer application rates and livestock densities. In a first step we applied the model to the global scale in combination with a randomization approach to assess the effects of LU on global native area-of-habitat for each of 5,200 mammal, 10,498 bird, 5,522 amphibian, and 5,730 reptile species analyzing the contribution of area extant and land use intensity. We calculated global gridded maps (5 arcminutes), depicting the number of terrestrial vertebrate populations lost (or impending to be lost due to time lags) in each grid cell due to biomass extracted by land use category, distinguishing 141 product types. We denote the sum of lost populations of vertebrate species across all grid cells as Biodiversity Footprint (BDF) of a biomass product. The model and its application for analyzing global vertebrate species loss was published in Nature Communications (Semenchuk et al., 2022).
Extending the initial project plan beyond the focus on terrestrial biodiversity impacts, we used data on nitrogen fertilizer (synthetic and manure) use per crop to assess marine eutrophication caused by crop production and consumption (Bidoglio et al., 2023a). Specifically, we investigated how production and trade of crop products drive oxygen depletion in coastal systems, one of the biggest threats to marine biodiversity. We coupled data on global production and nitrogen fertilization for 152 crops with a life cycle assessment-based model on oxygen depletion impacts. We first estimated the extent of oxygen depletion occurring in 66 Large Marine Ecosystems due to agricultural production in the watersheds draining into them. We then combined this with data on global trade and consumption of crop products. We show that, unsurprisingly, impacts are especially large in systems where adjacent land systems are used intensively and where mixing of coastal water bodies is limited (e.g., Balic Sea, Bay of Bengal, South China Sea). We also show that in many contexts, current trade patterns actually lower overall pressure on marine systems compared to a no-trade counterfactual. In a follow-up study, we investigated how livestock products drive environmental pressures, contrasting the impacts on marine eutrophication with contributions to terrestrial biodiversity loss and ongoing deforestation (Bidoglio et al., 2023b). This pioneering research provides the basis to extend BDF indicators to capture impacts of (urban) biomass use on marine ecosystems in future research.
Vienna’s biomass metabolism
To quantify the biodiversity footprint of biomass use in the city of Vienna we analyzed the urban biomass metabolism, accounting for the mass of biomass-products used as food, energy or in material applications within the city. We developed a mass balanced material flow model to convert final consumption of biomass products into primary biomass requirements and which traces primary biomass to the country of origin (Kalt et al., 2021). Based on this model we were able to link biomass consumption in the city to spatially explicit global land use maps. Since data on the consumption of food and other biomass products specifically for Vienna are largely lacking, we first analyzed biomass flows through the Austrian economy and in a second step downscaled consumption data to the Viennese scale.
We find that in 2010, the city of Vienna’s 1.7 million inhabitants used 1.76 MtDM biomass as food, energy carrier and various materials. To produce these commodities, 3.21 MtDM primary biomass were extracted from terrestrial ecosystems worldwide, which implies that roughly 1.45 MtDM primary biomass were lost during conversion processes or as waste flows. To extract the total of 3.21 MtDM primary biomass, about 14500 km² of land was converted and used in various ways in Austria, Europe and the global hinterland, which is roughly 35 times the area of Vienna itself. Figure 1 shows biomass consumption in Vienna and embodied primary biomass broken down by use categories and product groups. While in terms of product mass, material products account for the largest fraction (45 %), primary biomass is clearly dominated by food (59 %). Although Austria’s largest bioenergy plant is located in Vienna (the combined biomass heat and power plant Simmering), biomass for energy accounts for a significantly smaller fraction of total biomass use in Vienna than in the whole of Austria (13 % and 43 % of the total primary biomass, respectively). The fuel consumption of the biomass plant Simmering makes up about half of the wood fuel consumption in Vienna. The rest is mainly log wood used for residential heating in small-scale boilers and stoves. Liquid biofuels, mainly biodiesel and ethanol, represent 4.5 % of all biomass products consumed in Vienna. Material use accounts for 28% of embodied primary biomass and is dominated by biomass for wood and paper products (35% and 32% of primary biomass in material use).
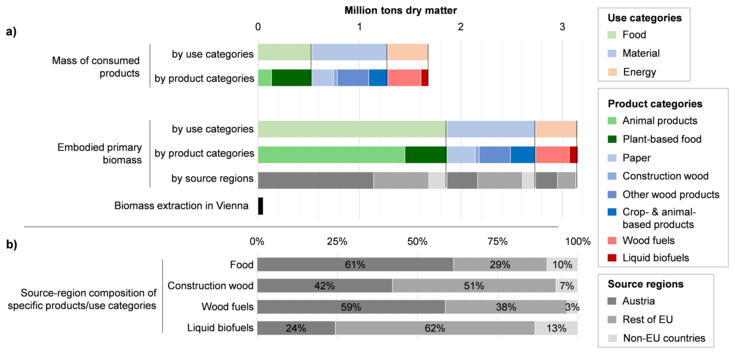
Figure 1: Biomass flows in Vienna (average 2010-2013). Biomass consumption in Vienna by use categories, product groups and source regions (1a). Composition of primary biomass for food, construction wood and fuels by source regions (1b). Source: Plank et al. (2023).
Vienna is a city with a high share of green space (45%). Although most of this land is under some sort of conservation regime (including a National Park and Biosphere Reserve) a considerable fraction is also used for biomass extraction including ca. 3500 ha of cropland and vineyards. The biomass harvested on this land amounts to only 2% of Vienna’s primary biomass demand and only part of it is actually consumed in Vienna. Despite Austria’s large forest areas and a high degree of apparent self-sufficiency with agricultural and animal products, biomass consumed in Vienna is to a large degree sourced from foreign countries. Other EU countries account for the largest part of primary biomass embodied in Vienna’s consumption, but imports from non-EU countries are also of relevance, particularly for food supply and liquid biofuels. While feed supply for ruminants in Austria can be predominantly ensured by biomass from domestic grassland, products from monogastric animals show a large share of biomass from abroad. These imports compensate for a lack of protein feedstuff (primarily soy) from domestic agriculture for prevailing animal production levels and originate mainly from countries like Brazil, Argentina or the USA. A main contributing factor to the high share of imported material in Vienna’s wood consumption is that the Austrian wood industries are importing large quantities of roundwood, processing it to semi-finished and final wood products, and exporting large shares of their production. Thus, wood products consumed in Vienna, as well as wood processing residues being used for energy, contain large shares of imported material. The model and the analysis of biomass flows in Austria has been presented in Kalt et al. (2021); results for Vienna are shown in Plank et al. (2023) and Sementschuk et al. (2023).
Vienna’s biodiversity footprint
To calculate the biodiversity footprint of biomass consumed in Vienna we established gridded maps of the area requirements for Vienna’s biomass consumption. For this calculation, we matched the 133 primary biomass products distinguished for Vienna to 45 LU-types available in the cSAR model. In absence of information on the exact locations that serve Vienna’s consumption within a given country, we assumed that the distribution proportionally follows the distribution of biomass production for the considered product and LU-type combination in the target country (excluding areas predominantly used for subsistence production). By combining the maps representing land use for consumption in Vienna and land use specific species loss information derived from the global cSAR model (characterization factors) we were able to produce spatially explicit maps of Vienna’s biodiversity footprint shown in Figure 2. We find that to produce the 3.21 MtDM primary biomass required for the consumption of food, energy and materials in Vienna 14500 km² of land is used in various ways in Austria, Europe and the global hinterland, which is roughly 35 times the area of Vienna itself. Biomass extraction from these areas resulted in impending losses of 10905 populations (“population” here denotes the disappearance of a species from a grid cell of 5 arcmin size; see above) of mammals, birds, reptiles and amphibians world-wide, representing the total Viennese biodiversity footprint (BDF). Of the total global BDF of the city of Vienna, about 58% were caused by food, 13% by energy and 28% by material consumption. About 43% of this footprint was caused by the consumption of animal products (both food and non-food). The LU-types forest and timber plantation cause about 11 and 17% of this footprint, pastures and grazing land about 16% and 2%, and the total sum of permanent and annual crops about 54%, respectively.
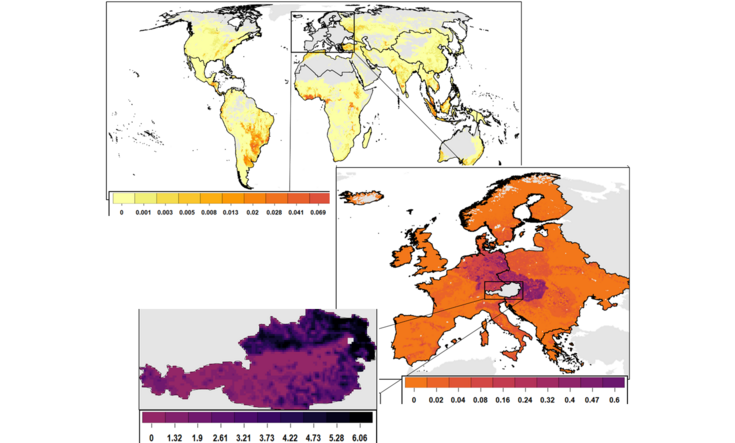
Figure 2: Total biodiversity footprint of each 5 arcmin cell (maps). Maps show terrestrial vertebrate populations impending to be lost due to the biomass consumption in the city of Vienna, Austria. Note the different scales across panels which include (2a) the world without Europe, (2b) Europe without Austria, and 2(c) Austria only, respectively. The sum across all grid cells equals the total biodiversity footprint of Vienna, which comprises vertebrate species loss in 41 land-use-types embodied in 43 products. Source: redrawn from Semenchuk et al. (2023).
We found large differences in BDF between products. Not surprisingly, the impact of animal products on species loss is highest, in particular for beef and mutton which have low conversion efficiencies and are land intensive. In general, the BDF of most animal products is roughly an order of magnitude higher than for most other food products. Also, stimulants and spices, mainly imported from tropical regions, have high per quantity BDFs because of low yields (i.e. area needed per quantity) and their origin in regions with high native species richness. For example, coffee, which only accounts for 0.3% of biomass consumption in Vienna causes 3% of the city’s BDF. Roots and tubers, and sugar have the lowest impact per product quantity due to high yields and conversion efficiencies to final products. Interestingly, products from cereals and wood, which are consumed in highest quantities, have a similar and comparatively low relative biodiversity impact. The product-specific BDFs we calculated are specific for Vienna (and Austria); they may differ considerably for other cities, which source their products from other regions with different production systems and native species richness. The results of the quantification and analysis of the BDF of Vienna for the base year 2010 have been published in Semenchuk et al. (2023).
Scenarios for reducing the BDF of Vienna
In the scenario analysis we focused on food system changes, since the food system was responsible for nearly 60% of the species loss caused by biomass consumption in Vienna. We investigated the differences in biodiversity loss associated in 24 alternative food consumption scenarios varying in diet, calorie intake and levels of food waste; additionally, we analysed the effect of shifting from imported to domestically (in Austria) produced food. Not surprisingly, we find that reductions of Vienna’s food-related biodiversity impact can primarily be achieved by reducing the consumption of resource-intensive animal products. Dietary shifts towards vegetarian or vegan diets can reduce Vienna’s biodiversity impact by up to 37 and 43%, respectively. Shrinking the livestock sector would not only benefit biodiversity, but has an even larger potential to decrease GHG emissions (Lauk et al., 2022), due to the high impact created by methane produced by ruminants during enteric fermentation.
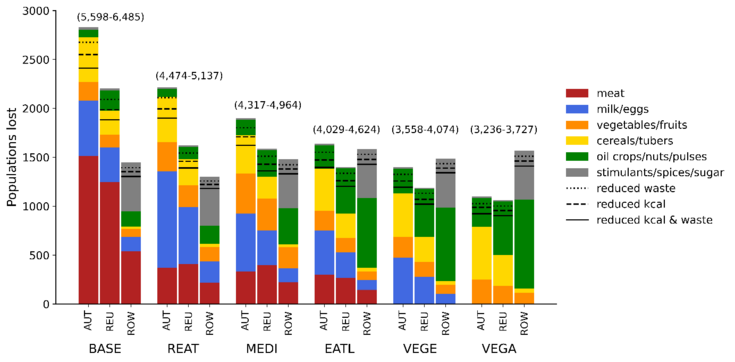
Figure 3: Biodiversity loss under 24 alternative food consumption scenarios within Austria (AUT), the rest of Europe (REU) and the rest of the World outside Europe (ROW), attributed to the consumption of various products. Scenarios encompass the Baseline diet (BASE) and 5 diet variants for high and reduced demand levels, respectively, under the assumption of current trade patterns (proportional production). The range of global biodiversity loss totals (populations lost) for high and reduced demand levels is given above bars per diet. Source: Matej et al. (2023).
Compared to diet change, demand reductions have a lower potential to decrease biodiversity losses in absolute terms. Decreasing the demand by adhering to the recommended calorie intake of 2,614 kcal/cap/day can reduce biodiversity losses by 9% per diet, and would also reduce GHG emissions and benefit public health (Magkos et al., 2020). Waste reductions are broadly discussed as a measure to promote food security and decrease environmental impacts (Beretta and Hellweg, 2019; Scherhaufer et al., 2018). For Vienna we found that halving avoidable waste at the retail, food service and household level in Vienna can decrease biodiversity impacts by only up to 5% per diet, due to only 9% of the baseline calorie supply being avoidable waste in Austria (Lauk et al., 2022). This contrasts with findings for the USA, where equivalent food waste reductions were found to reduce biodiversity losses by 17.5% (Read et al., 2022). Our estimate corresponds with the lower end of the range (5 to 17%) given by Helander et al (2021) for potential biomass footprint reductions through halving food waste for Germany’s current diet. Since our analysis has shown that the BDF of a specific product can vary significantly depending on the production region, we also explored the potential for biodiversity loss reduction through relocation of production to the country of consumption. For the case of Vienna and the different alternative food consumption scenarios, this can reduce total biodiversity losses by 4.5 to 21.4% (compared to proportional production changes), due to often higher yields and lower native species richness in Austria than in other countries. While relocation can reduce Vienna’s total biodiversity impact, it diminishes the benefit for biodiversity within Austria that could be achieved by diet change and demand reduction without changing production patterns.
Besides animal products, coffee and cocoa also play a major role in food related biodiversity impacts, due to both low yields and high biodiversity loss factors. With their current consumption accounting for 8% of the Viennese biodiversity impact, changing demand levels for stimulants could contribute significantly to reducing biodiversity losses. However, they are often excluded from dietary recommendations or scenario analysis. Another issue are products like nuts, which, in the EATL, VEGE and VEGA diets are an important substitute for animal products, which would increase Vienna’s demand for nuts more than tenfold. Although this substitution can decrease biodiversity losses for Vienna, concerns have been raised regarding the feasibility of upscaling nut production to meet the EAT-Lancet recommendations on a global scale and its effects on water use (Vanham et al., 2020). A manuscript by Matej et al. (2023) presenting the results of the scenario analysis has recently been submitted to Science of the Total Environment.
Governing the spatial mismatch of urban biomass consumption and its impact on biodiversity
We conducted interviews with relevant local stakeholders to explore current and potential governance options and a workshop with stakeholders to discuss options to reduce the BDF of Vienna. We focused on the three sectors food, bioenergy and timber use in construction and specifically explored issues related to the spatial mismatch between urban consumption and the supply regions in the regional and global hinterland and implications for governance instruments. As outlined in Plank et al. (2023), we found that the spatial mismatch and the hidden character of the value creation process make it difficult for actors at the city level to exert influence on the BDF of the biomass consumed. Although the City of Vienna offers a variety of activities which foster a “direct approach” to biodiversity conservation within the city, this only regards a small amount of biomass produced and consumed in Vienna and ignores impacts in the global hinterland. The two fields of action for governance of biomass consumption at the urban level which the interviewees have named are public procurement and energy generation in the biomass plant. At the city scale in particular public procurement and the promotion of consumption patterns with lower land and primary biomass demand appear as important leverage points. An important prerequisite is unambiguous information and labelling concerning the biodiversity impacts of biomass products which is complicated by the fact that indirect land use effects need to be considered. Currently, none of the governance measures investigated includes explicit biodiversity criteria. To effectively address the spatial mismatch, a different mode of production – regulated at national and international scales and including mandatory measures – and a different mode of consumption as well as a reduction of consumption and production is required. Information like that provided by product specific biodiversity footprint indicators can support decision making by urban stakeholders.
References:
Beretta, C., Hellweg, S., 2019. Potential environmental benefits from food waste prevention in the food service sector. Resources, Conservation and Recycling 147, 169–178.
Bidoglio, G., Mueller, N.D., Kastner, T., 2023a. Trade-induced displacement of impacts of global crop production on oxygen depletion in marine ecosystems, revised version submitted to Environmental Research Letters.
Bidoglio, G., Schwarzmueller, F., Kastner, T., 2023b. A global multi-indicator assessment of the environmental impact of livestock products, manuscript in preparation.
Helander, H., Bruckner, M., Leipold, S., Petit-Boix, A., Bringezu, S., 2021. Eating healthy or wasting less? Reducing resource footprints of food consumption. Environmental Research Letters 16, 054033.
Kalt, G., Kaufmann, L., Kastner, T., Krausmann, F., 2021. Tracing Austria’s biomass consumption to source countries: A product-level comparison between bioenergy, food and material. Ecological Economics 188, 107129.
Lauk, C., Kaufmann, L., Theurl, M.C., Wittmann, F., Eder, M., Hörtenhuber, S., Freyer, B., Krausmann, F., 2022. Demand side options to reduce greenhouse gas emissions and the land footprint of urban food systems: A scenario analysis for the City of Vienna. Journal of Cleaner Production 359, 132064.
Magkos, F., Tetens, I., Bügel, S.G., Felby, C., Schacht, S.R., Hill, J.O., Ravussin, E., Astrup, A., 2020. A perspective on the transition to plant-based diets: a diet change may attenuate climate change, but can it also attenuate obesity and chronic disease risk? Advances in Nutrition 11, 1–9.
Matej, S., Kaufmann, L., Semenchuk, P., Dullinger, S., Essl, F., Haberl, H., Kalt, G., Kastner, T., Lauk, C., Krausmann, F., Erb, K.H., 2023. Options for reducing a city’s global impact on biodiversity – The case of food consumption in Vienna, manuscript submitted to Sustainable Production and Consumption.
Newbold, T., Hudson, L.N., Hill, S.L., Contu, S., Lysenko, I., Senior, R.A., Börger, L., Bennett, D.J., Choimes, A., Collen, B., 2015. Global effects of land use on local terrestrial biodiversity. Nature 520, 45–50.
Pereira, H.M., Ziv, G.U.Y., Miranda, M., 2014. Countryside species–area relationship as a valid alternative to the matrix-calibrated species–area model. Conservation Biology 28, 874.
Plank, C., Görg, C., Kalt, G., Kaufmann, L., Dullinger, S. & F. Krausmann, 2023. “Biomass from somewhere?” Governing the spatial mismatch of Viennese biomass consumption and its impact on biodiversity. Land Use Policy 131, 106693.
Read, Q.D., Hondula, K.L., Muth, M.K., 2022. Biodiversity effects of food system sustainability actions from farm to fork. Proceedings of the National Academy of Sciences 119, e2113884119.
Scherhaufer, S., Moates, G., Hartikainen, H., Waldron, K., Obersteiner, G., 2018. Environmental impacts of food waste in Europe. Waste management 77, 98–113.
Semenchuk, P., Kalt, G., Kaufmann, L., Kastner, T., Matej, S., Bidoglio, G., Erb, K.-H., Essl, F., Haberl, H., Dullinger, S., 2023. The global biodiversity footprint of urban consumption: A spatially explicit assessment for the city of Vienna. Science of The Total Environment 861, 160576.
Semenchuk, P., Plutzar, C., Kastner, T., Matej, S., Bidoglio, G., Erb, K.-H., Essl, F., Haberl, H., Wessely, J., Krausmann, F., 2022. Relative effects of land conversion and land-use intensity on terrestrial vertebrate diversity. Nature communications 13, 1–10.
Vanham, D., Mekonnen, M.M., Hoekstra, A.Y., 2020. Treenuts and groundnuts in the EAT-Lancet reference diet: concerns regarding sustainable water use. Global food security 24, 100357.