Impact of mass transfer mechanism on protein separation in two-component adsorption
SUPERVISOR: Rainer HAHN
PROJECT ASSIGNED TO: Jürgen BECK
1. Project description
Designing a protein chromatography process requires an understanding of the rate at with which proteins bind to the chromatography media. The protein adsorption rate is usually controlled by diffusion inside the particles. Intraparticle mass transport is based on pore diffusion, solid-phase diffusion, or a combination of both[1]. There is a variety of different resins with diverse stationary phase characteristics available which directly influence the mass transport of the protein species[2]. The dominant driving force depends on the composition of the adsorbent, the properties of the respective proteins as well as the operating conditions, e.g. buffer composition. Recent studies on two- or multi-component mixtures and their adsorption properties based on one or the other mechanism have mostly been restricted to protein variants or only one type of resin[3–5].
The core of the PhD thesis will be the investigation of adsorption and desorption processes of two-component systems comprising model proteins of different properties and sizes. The intraparticle mass transport will be evaluated using resins with four distinct types of solid phase characteristics inside the pore space. The impact of the type of mass transport on the adsorption and desorption rate or competitive binding effects of the two-component mixtures on the respective media will be investigated using established experimental techniques
In this doctoral thesis the cation exchange resins SP Sepharose FF, Capto S, Capto S ImpAct and S HyperD will be compared. Each resin has a unique pore structure. SP Sepharose FF has “smooth” pores ligands are covalently attached without using grafting techniques. Capto S and Capto S ImpAct both have covalently attached polymers on the inside of the pores, increasing the surface area, and therefore ligand density and binding capacity. For Capto S ImpAct acrylamide polymer chains containing the functional group (ligand) are attached at the chain ends on the resin forming a tentacle-like structure inside the pore, which results in a so-called single-point attachment. In the case of Capto S, a dextran polymer with the functional group is introduced into the resin and bound covalently via a multi-point attachment mechanism. The main difference between the types is the chemical structure and the type of attachment to the resin[6]. S HyperD is a composite resin comprising rigid backbone of polystyrene coated silica and a pore space filled with polyacrylamide-based hydrogel.
The impact of the intraparticle structure of these resins on the mass transport and sorption processes will be investigated thoroughly.
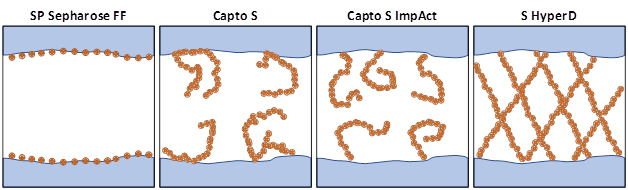
Figure 1: Schematic of the structure of the types of functionalization in pores of resins used in this thesis
First, the transport and adsorption parameters will be determined for single component systems. Model proteins of different sizes and properties will be used, e.g. lysozyme, cytochrome c, antibodies and possibly others. The experiments will include adsorption isotherms, batch uptake kinetics, breakthrough curves and elution experiments.
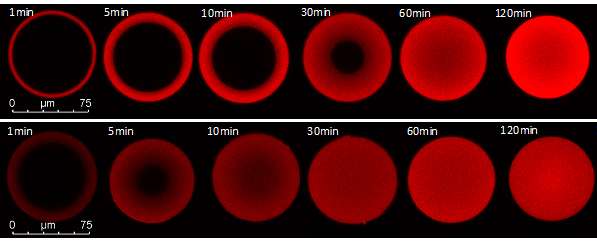
Figure 2: CLSM images of monoclonal antibody adsorption and comparison of different mass transport mechanisms. Top: High binding strength on resin with pore diffusion transport conforms to the so-called shrinking core model. Bottom: High binding strength in a resin with apparent solid diffusion transport is characterized by diffuse boundary layers and faster transport.
Subsequently, two-component systems of different compositions will be tested in the same way. Buffer conditions will be varied to investigate transport of the proteins at weak binding strength and gain insight on the transport. The adsorption process over time can be visualized using confocal laser scanning microscopy (CLSM) with labeled proteins. Additionally, mechanistic modeling will be used to gain an understanding of the intraparticle transport processes. Particularly the difference between solid-phase diffusion and pore diffusion at relatively weak binding strength will be worked on theoretically in addition to the experiments. Predictions of the mechanistic model at the particle level will be evaluated and compared to experimental results obtained by CLSM.
2. References
1. Carta G, Jungbauer A. Protein chromatography: process development and scale-up. John Wiley & Sons; 2010.
2. Staby A, Nielsen J, Krarup J, Wiendahl M, Hansen T, Kidal S, et al. Advances in Resins for Ion-Exchange Chromatography. Advances in Chromatography. 2009 Feb 23;47:193–245.
3. Lewus RK, Carta G. Binary protein adsorption on gel-composite ion-exchange media. AIChE Journal. 1999;45(3):512–22.
4. Lewus RK, Carta G. Protein diffusion in charged polyacrylamide gels: Visualization and analysis. Journal of Chromatography A. 1999 Dec 31;865(1):155–68.
5. Lewus RK, Carta G. Protein Transport in Constrained Anionic Hydrogels: Diffusion and Boundary-Layer Mass Transfer. Ind Eng Chem Res. 2001 Mar 1;40(6):1548–58.
6. Lenhoff AM. Protein adsorption and transport in polymer-functionalized ion-exchangers. Journal of Chromatography A. 2011 Dec 9;1218(49):8748–59.